A page from the "Causes of Color" exhibit...
Light from objects in space

The sun, moon, and stars have been a source of fascination since early times, inspiring our curiosity, a host of legends, and even predictions about the future. With the advantage of modern sky-gazing equipment to observe not only the light, but also the background radiation that reaches us from deep space, we can see back in time almost to the birth of the universe. The night sky is a treasure of information about the history of our cosmos, and indeed provides clues as to the future of our sun, our galaxy, and the universe itself.
We can learn much about other stars by understanding our sun. |
This galactic “dust bunny” is known as a Bok globule. These concentrations of gas and dust tend to draw in more content from nearby stars and nebulae. If they gather enough mass, they can go on to form new stars. This Bok globule is located close to Cassiopeia, 9,500 light-years away. |
Light sources in the night sky include stars, galaxies, nebulae, and meteors. In addition to visible light, there are also invisible electromagnetic radiation signals reaching our atmosphere, such as ultraviolet light, infrared waves, microwaves, x-rays, and radio waves. Astrophysicists use this information to work out the structure of the universe, and discover how it behaves.
There are over 100,000 absorption lines in the sun’s spectrum. These enable us to analyze the sun’s composition.
Astronomical spectroscopy
Most of us are familiar with the rainbow of colored light that appears when sunlight is separated into its constituent wavelengths by a prism. A very sharp and bright image of this spectrum reveals another facet of sunlight: there are dark lines that form where wavelengths have been absorbed. These dark lines, known as an absorption spectrum, provide information about the chemistry of the sun.
Every chemical element has its own spectral “fingerprint,” a pattern of light across the visible spectrum that is evident in chemical flame tests. The structure of a substance at its atomic level defines very specific differences in energy between the allowed energy states of its electrons. These energy differences in turn correspond to specific wavelengths when the substance gains or loses energy as photons of light.
There are two kinds of spectra. Line emission spectra consist of individual lines of light emitted by an element or combination of elements. Absorption spectra appear as dark lines in the visible light spectrum, absorbed from incident light by particles of gas or dust between the light source and the observer.
An important consideration for astronomical spectroscopy is the correction for red shift (or blue shift), a change in the wavelength, and hence the color, of light that has traveled over long distances to reach us.
The bottom spectrum is the absorption spectrum of the sun, and those above it for galaxies progressively further away. The pattern of absorption lines shifts further and further to the right, toward the red end of the spectrum.
Red shift: the Doppler effect in light
Light that reaches us from the farthest parts of the universe doesn’t appear to us as it did at its source. Just as the Doppler effect accounts for the changing pitch of a passing fire truck as it approaches and then moves away from us, moving light changes in frequency too. The frequency is inversely proportional to the wavelength, so that as the frequency decreases, the wavelength increases. Just as sound distorted in this way changes pitch, light changes color.
Where the Doppler effect increases wavelength for objects moving away from the observer, visible light shifts towards the red end of the spectrum. This is known as red shift, even if the wavelengths involved are outside the visible light range. A light source moving towards the observer increases in wavelength, and as this takes visible light toward the blue end of the spectrum, it is known as blue shift.
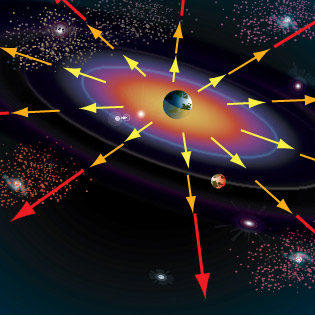
According to cosmic expansion theory, the universe expands uniformly, creating the illusion that we are at its center as everything moves away from us. The further light has travelled to reach us, the greater the red shift as its wavelength is distorted to higher values.
It turns out that the light reaching us is predominantly red-shifted. Understanding this red shift is a powerful endorsement of the theory of cosmic expansion. In this model, our universe is expanding outwards uniformly. By analyzing the light from an object, and measuring the extent to which it has been distorted by red shift, we are able to estimate the rate of expansion using Hubble’s Law: recessional velocity as determined from red shift is proportional to distance (for distant objects).
BWorking back using this rate of expansion, we can estimate the age of the universe. This means we are effectively looking back in time, looking at light that was emitted in the early days of the universe.
In the early 20th century, Georges Lemaître proposed what became known as the Big Bang theory. The theory states that if the universe is expanding, it must have started as a single point. He said that this event would have left behind some signature radiation. Some time later, scientists began to look for corroboration of this model, theorizing that light from the beginning of the universe would be red-shifted to microwave wavelengths.
They were scooped in the 1960s by two researchers who were trying to eliminate the noise background picked up by microwave antennae. Unable to get rid of a mysterious background signal that remained constant in any direction, at any time, Arno Penzias and Robert Wilson phoned Princeton University, reaching the team that was looking for this very type of radiation.
At present, cosmologists are very interested in small variations in the cosmic microwave background and these are proving a rich source of information. The Planck mission, using a satellite launched on 14th May 2009, aims to measure these variations more accurately than has been achieved before. This may yield vital clues as to the distribution of dark energy and other open questions.
The Doppler effect has also enabled astronomers to identify planets that are too faint to be seen. A visible star and the unseen planet orbiting it both orbit a common center of gravity. The "wobbles" in the star’s orbit allow the observer to deduce the mass and the orbit of the planet. To do this, they make use of instrumentation sensitive enough to pick up the red shift of light moving at just 1 meter per second.